
In this section you will learn how the Hammond postulate helps you understand the relationship between kinetics and thermodynamics in organic reactions. Before we consider the postulate, let's begin by considering a reaction in which two possible products can be formed by the same starting material:
If product A is the major product, we can say that product A is either a more stable product, or it formed at a faster reaction rate. However, there are two factors needed to be taken into account: thermodynamics (more stable) and kinetics (forms faster). The relative energies of the two products are shown in Figure 1.4.
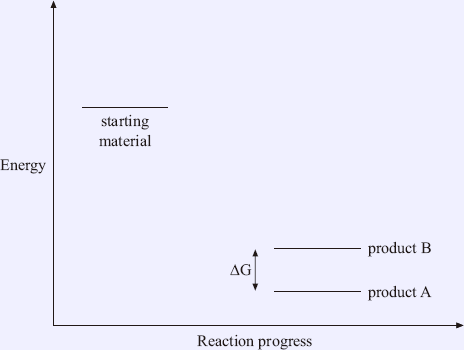
Another diagram (Figure 1.5) shows the formation of a more stable product A in a transition state with a lower energy than that of product B. The activated energy required for the reaction pathway for product A is smaller. Hence, product A can be obtained faster as a more stable major product in this case.
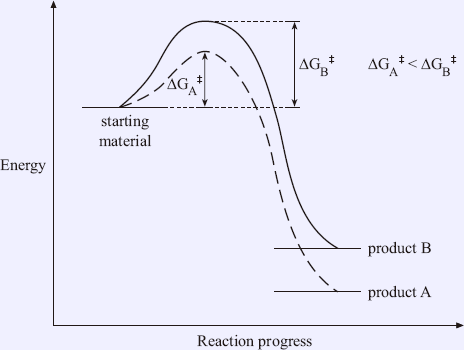
Although product A has lower energy, it will not necessarily form faster than product B. It is possible for the more stable products to have a transition state with higher energy (Figure 1.6). In this case, product B will form faster even though it is the less stable product.
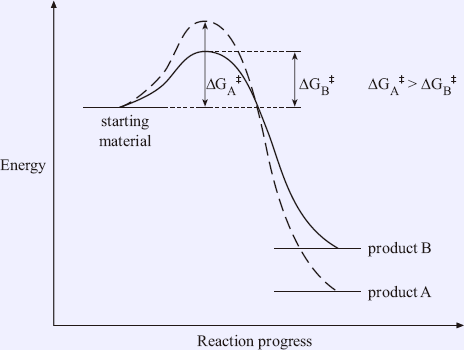
In such cases, the major product of this reaction depends on which factor will win out: thermodynamic control or kinetic control.
If there is enough energy to overcome all the reaction barriers for both products, and the reaction is in equilibrium with the one with lower energy that is more stable, this reaction is regarded as thermodynamic control. The ratio of products depends on the ΔG between the two products A and B. On the other hand, if the reaction tends to produce a less stable product as a major product, and the activation energy for this reaction pathway is smaller with a lower transition state, the reaction can be considered as kinetic control. The ratio of products depends on the relative difference between their activation energies.
In a reaction free-energy diagram, the transition state has a maximum energy relative to both the reactants and products. It is unstable and cannot be isolated; however, you can imagine that the transition state is a structure that exists somewhere between the reactants and the products, and that all reactions should possess this stage. Usually, the transition involves the process of bond breaking and bond forming. These partial bonds are shown in dashed lines.
For example, in the addition of HCl to 2-methylpropene, the transition state of the first step (formation of carbocation) is shown in Figure 1.7.
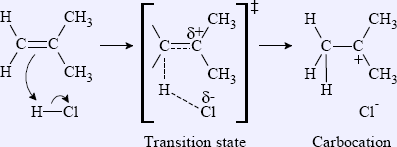
In the transition state, the π bond of the alkene and the σ bond of H–Cl are breaking, while a C–H σ bond is forming. These partial bonds are represented in dashed lines, with the partial charges δ+ and δ–, which means partial positive and partial negative, respectively. The final product in this reaction is the carbocation and chloride ion with full charges. The major factor that affects the instability of the transition state is the charge separation of the positive and negative charge, and the development of the electron-deficient site.
For the above example, 2-methylpropene is an unsymmetrical alkene which can be protonated at either side of the double bond to give two different carbocations. The free energy diagram is shown in Figure 1.8.
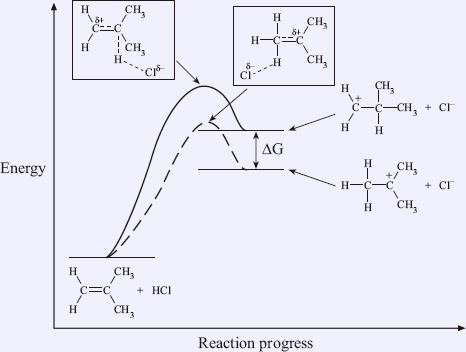
The two carbocations have different stabilities. The tertiary carbocation with more substituted (i.e. the lower energy one) is more thermodynamically stable than the primary carbocation (i.e. the higher energy one). This is because the substituents stabilize the positive charge on the carbocation by positive inductive effect. The factors that stabilize a full positive charge can be operated to stabilize a partial positive charge. Hence, the transition state for the formation of a tertiary carbocation is more stable than that of a primary carbocation.
An approximation with their structural similarity assumption can be made. This is called Hammond's postulate, which is named for George. S. Hammond, who first stated and used it in organic reactions in 1955.
Hammond's postulate
Assume that the transition states for reactions involving unstable intermediates can be closely approximated by their intermediates.
This means that we can assume the structures of transition states are very similar to those of their intermediates. Since the tertiary carbocation is more stable than the primary carbocation, the transition state leading to the more stable tertiary carbocation should have lower energy, as predicted by Hammond's postulate. Hence, protonation of 2-methylpropene to give a tertiary carbocation proceeds in a pathway with lower activation energy. Therefore, it can be produced at a faster reaction rate.
A complete free energy diagram for the two possible reaction pathways of addition of HCl to 2-methylpropene is shown in Figure 1.9. It should be noted that the stabilities themselves cannot determine which pathway is faster. Only making use of Hammond's postulate can allow us to relate the carbocation energy and transition state energy, and then use the concept of activation energy to determine which one is faster.
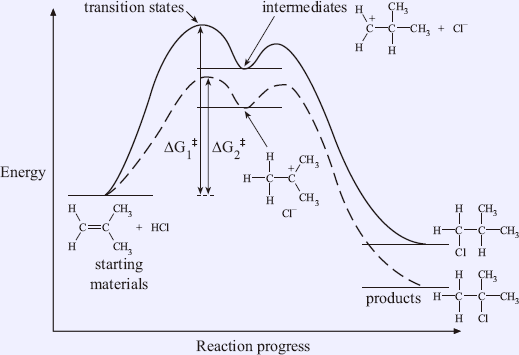
In the above example, the formation of tert-butyl chloride is the fastest because it involves the formation of the most stable carbocation, and its transition state should have the lower energy. It then requires less activation energy. Although the structures in transition states are not certain, the structures of the starting materials, products and intermediates can be used in Hammond's postulate, and we can assume that the transition states and their corresponding reactive intermediates have similar structures.
- 11740 reads